Introduction
Rapid urbanization has brought urban air quality problems, including particulate pollution, worldwide (Hopke et al., 2008). Exposure to urban particulates has adverse effects on human health, especially on the cardiopulmonary system (Dockery et al., 1993; Kumar et al., 2007; Li et al., 2015). Humans can be exposed to heterogeneous mixtures of particles emitted from various sources. In addition, exposure to PM can disrupt the equilibrium between cell death and regeneration, which affects organismal homeostasis (Peixoto et al., 2017). Although PM is only one of several factors causing aggravation of human health, numerous epidemiological studies have reported both short and long-term exposure to PM can result in adverse effects on the respiratory and cardiovascular systems (Brook et al., 2010; Kim et al., 2014; Evans et al., 2017).
The atmospheric PM also called aerosol particles or particulates (Figure 1) is a suspended mixture of solid particles and liquid droplets in the air (Pöschl, 2005; Falcon-Rodriguez et al., 2016). PM is a heterogeneous mixture of particles differing in size, source, and chemical composition. Atmospheric aerosol particles range from 1 nm to 100 μm in aerodynamic diameters (dp). The United States Environmental Protection Agency (US EPA) classified PM by different size fractions according to human inhalation toxicology for regulatory purposes (particulates with a dp ≤10 μm as PM10 and a dp of ≤2.5 μm as PM2.5) (Burkhardt, 2010; Kurth et al., 2014; Burkhardt and Grantz, 2016; Falcon-Rodriguez et al., 2016).
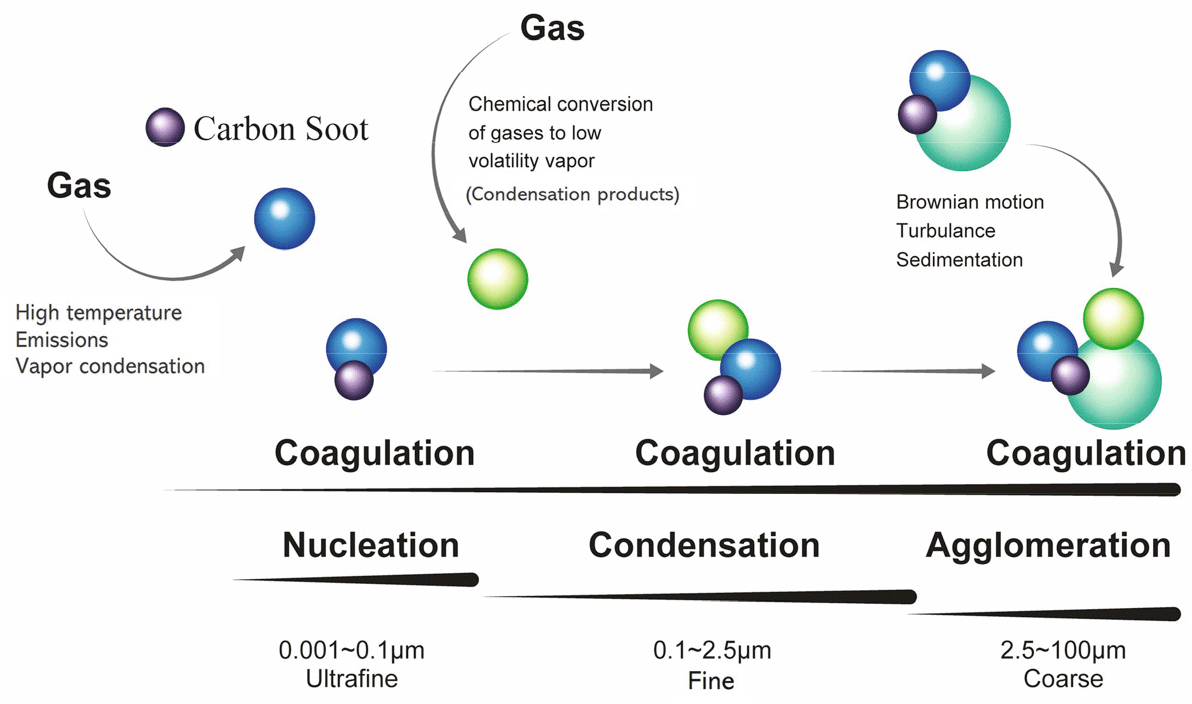
With 68% of the world’s population projected to live in cities by 2050 (UN, 2018), urban particle pollution is rapidly emerging as one of the most critical environmental problems in many parts of the world (Chen et al., 2015). Urban greenery can widely function as a biological filtration that intercepts airborne PM. Previous studies have demonstrated that PM capturing and retaining capacities on leaves depend on surface roughness and microstructure properties based on leaf macro- and micro-morphology, anatomy, and chemistry (Chen et al., 2017; Kwak et al., 2020; Zhang et al, 2020). Therefore, a better understanding of the underlying physical processes between deposition and wash-off of PM particles on leaf macro- and micro-morphological variability is important to the potential merits of urban greenery to bolster urban air pollution control.
Urban green space is broadly defined in the current study as “any vegetation found in the urban environment, including parks, open spaces, residential gardens, or street trees” (Kabisch and Haase, 2013). The green infrastructure consists of all types of vegetation in urban areas, such as street trees, vegetation barriers (including roadside hedges), and green walls/roofs; in particular, trees are used as an effective tool to mitigate urban outdoor air quality, planted as part of the urban roadside landscape, in urban parks, and other extra spaces (Kumar et al., 2019). In urban environments, green space is considered one of the potential solutions to improve sustainability and alleviate air quality in response to the growing urban population (Salmond et al., 2016; Abhijith et al., 2017). The mechanism, process, and degree of fine dust reduction by green spaces are complex and attributed to various factors (Chen et al., 2019).
Prior investigations have demonstrated the PM capturing capacity of different plant species and identified which factors of leaves affect PM deposition on leaves (Sæbø et al., 2012; Liang et al., 2016; Chiam et al., 2019; Sgrigna et al., 2020; Zhang et al., 2020). Leaf characteristics affecting PM capturing ability could be divided into leaf morphology (macromorphology such as leaf size, shape, margin, and venation patterns and micromorphologies such as trichomes, stomata, and roughness) and physicochemical properties including leaf wax layer, which is attributable to surface wettability.
There are two techniques for quali-quantitative analysis of PM, using vacuum filtration or scanning electron microscopy with energy-dispersive X-ray spectroscopy (SEM/EDX) (Sgrigna et al., 2020). The washing/filtration procedure, also called separate gravimetric analyses, has been widely applied to quantify the mass of PM deposition on leaves in chemical fields (Dzierżanowski et al., 2011; Sgrigna et al., 2015; Song et al., 2015). The mass size distributions of PM can be measured by washing leaves with water or organic solvents such as chloroform and passing the solvents with PM through filters. This technique can reflect net aerosol because aerosols are removed from the atmosphere by resuspension, precipitation, and migration into the leaf by stomatal or cuticular uptake and deposited on the leaf surface via leaching from the leaf inside (Burkhardt and Grantz, 2016). The SEM/EDX is applied to measure the numerical density of PM adsorbed on leaf surfaces and identify the chemical composition of PM (Song et al., 2015; Baldacchini et al., 2017).
In this article, we first summarize the basic information on PM for a better understanding of this paper. We also review different topics regarding PM retention of urban greening species. We then offer the research evidence describing the relationship between leaf traits and PM retention for providing peer researchers and city planners to manage or design by utilizing urban greening as a sustainable method for PM reduction.
Data Source and Analysis
In this review, we analyze the bibliometric aspects of related studies on different scales of urban plant species for the removal efficiency of atmospheric particulate matter, as well as those addressing the particulate matter in general. We have also focused on the retrieval of information from quality scientific literature using the international bibliographic databases of Google Scholar from 1992 to 2021.
This study performed author keyword analysis to investigate scientific publications based on particulate matter research topics. The following keywords were searched to retrieve relevant articles: ‘urban greening’, ‘PM2.5’, ‘PM10’, ‘particulate matter’, ‘PM emission’, ‘PM biofilter’, ‘PM sources’, ‘PM capture capacity’, ‘PM wash-off’, ‘PM adsorption’, ‘leaf macromorphology’, ‘leaf micromorphology’, and ‘leaf physicochemical traits’.
Sources and Generation Mechanism of PM
The atmospheric PM originates from natural and anthropogenic sources such as wind erosion, volcanic eruptions, marine salt formations, suspension of biological materials (microorganisms, pollens, etc.), combustion of coal and fossil fuels, industrial production, fertilizer production, and vehicle emissions (Pöschl, 2005; Dzierżanowski et al., 2011; Wang et al., 2015). Primary particles are liquids or solids directly emitted from various sources. In contrast, secondary particles are formed by gas-to-particle transformation, including nucleation and condensation of gaseous precursors, i.e., NOx, NH3, SO2, and volatile organic compounds (VOCs) in the atmosphere (Pöschl, 2005; Fuzzi et al., 2015).
Atmospheric particles undergo aging and changing their size, physical structure, and chemical composition (Figure 2). As water vapor condenses on existing particles, PM acts as cloud condensation nuclei (CCN) and ice nuclei (IN). The modified particles can be released from the cloud droplets or ice crystals in reevaporating clouds. Then, through wet deposition, the primary mechanism of aerosols, particles are carried to the earth’s surface, interacting with airborne water particles (hydrometeors, i.e., rainfalls, sleets, hail). In addition to eliminating PM by precipitation, dry deposition, which occurs without hydrometeors and affects local air quality and human health, is another removal mechanism of particles from the air (Pöschl, 2005).
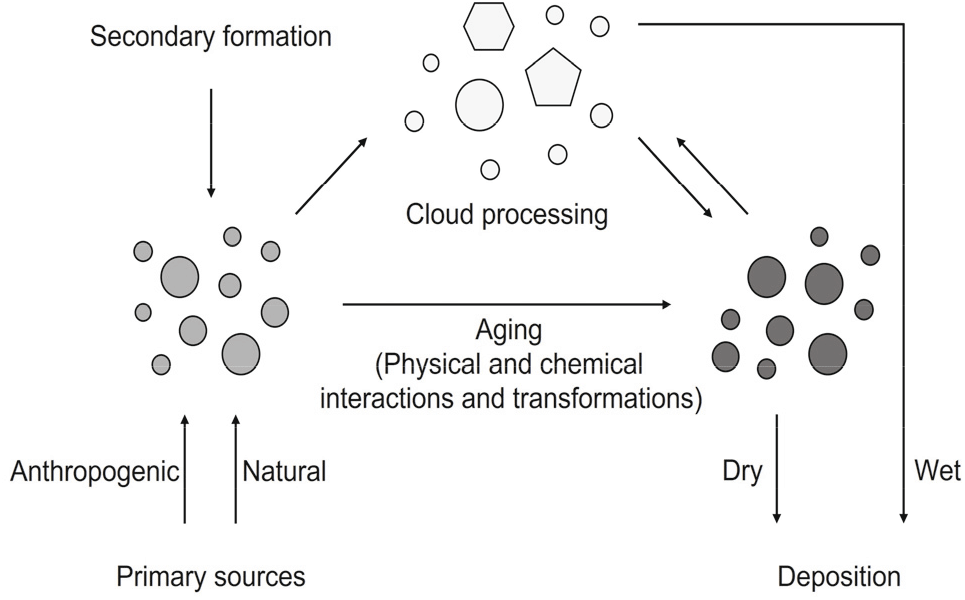
The major chemical components of PM are inorganic ions, such as nitrate (NO3−), sulfate (SO42−), and ammonium (NH4+), mineral dust from geological sources, sea salt, toxic metals, and carbonaceous materials including secondary organic compounds, elemental carbon and black carbon (Fuzzi et al., 2015; Gajbhiye et al., 2019). In addition, atmospheric particles can be bound with various heavy metals, such as Pb, Cd, Cu, Zn, and Hg, emitted from industrial processes and vehicles (Gajbhiye et al., 2019). Therefore, the composition of PM is highly variable depending on time and space (Kaneyasu et al., 1995; Sisler and Malm, 2000).
Urban Greenery as PM bio-filters
Urban green spaces influence the dispersion and deposition of PM (Janhäll, 2005), and the effect of green infrastructures on urban air quality has been extensively studied (Table 1). Previous studies relevant to the impact of urban plants on PM reduction have focused on the PM adsorption ability of different species on various sizes of particles and the factors influencing PM accumulation for selecting plant species effective in the adsorption and retention of atmospheric PM. For example, Sæbø et al. (2012) examined the PM accumulation of 22 trees and 25 shrubs in Norway and Poland. There were differences in the amount of PM adsorbed on leaf surfaces in different size fractions among species. Chen et al. (2017) reported that the groove area ratio and trichome density are important leaf traits for effective PM2.5 capturing. Importantly, leaves with trichomes and grooves on their surfaces retained PM more effectively than leaves with water-repellent behaviors (Kwak et al., 2019). Kwak et al. (2019) revealed that Ginkgo biloba can lead to repeated removal of particles by excellent water repellency on its leaf surfaces, indicating PM net removal by self-cleaning. Moreover, Sgrigna et al. (2020) analyzed the relationship between leaf traits and the amount of PM on leaf surfaces sampled from twelve tree species in an urban-industrial city in Italy and presented an accumulation index (AI) by scoring each leaf trait significantly influenced the PM adsorption.
As stated above, the micro-roughness of leaf surfaces such as trichomes, ridges, and grooves on broadleaved species can be used as an indicator of effectiveness for PM adsorption (Sæbø et al., 2012; Kwak et al., 2019; Sgrigna et al., 2020; Zhang et al., 2020). However, it is particularly necessary to upscale the PM adsorption from leaf to canopy level using the total leaf area and/or leaf area index (LAI). In more detail, Kwak et al. (2019) have demonstrated that PM adsorption per unit leaf area was high in Zelkova serrata. However, based on the total leaf area, Platanus occidentalis had a relatively high DBH compared to other tree species, resulting in the highest PM adsorption (95 to 104 g tree−1) among the studied tree species. However, P. occidentalis and G. biloba have been reported as tree species to disperse the main allergenic pollens among urban trees in Seoul, Korea, so it is necessary to consider issues such as pollens when constructing urban infrastructure.
Urban forests, encompassing trees, bushes, grass, soil, and water in urban areas, are part of the green infrastructure and offer ecosystem services, including air purification and microclimate mitigation (Hirabayashi, 2021). Several studies estimated the air purifying effect of urban trees on forest scale. For example, Yang et al. (2005) investigated that 2.4 million trees in the center of Beijing remove 1261.4 tons of air pollutants annually, and 61% of removed pollutants (772 tons) were PM10. McDonald et al. (2007) simulated the effect of urban tree planting on depositions of PM10 in two conurbations of the United Kingdom (UK) using a geographic information system (GIS). It was found that urban trees can remove PM10 concentrations by 7-26%.
Washing off PM by rainfall events is considered a cleaning process of leaf surfaces as a filter for PM. To examine the role of urban green spaces as long-term sustainable measures for particle reduction, some studies the effect of rainwater on PM retention of leaf surfaces. Xu et al. (2017) reported the differences among four urban plant species in PM adsorption before rainfall, maximum PM wash-off during the different rainfall intensities, and maximum PM retention on leaf surfaces after wash-off by rainfall events. Zhang et al. (2019) studied the quantity of PM reduction and removal rates of five urban greening species by simulating different rainfall regimes. A schematic diagram of the decontamination process between leaf roughness and self-cleaning during rainfall events showed in Figure 3. Rainfall mostly redistributes PM particles on smooth leaf surfaces, whereas the particles adhere to the surface of the droplets on rough leaf surfaces and are washed off the leaves (Barthlott and Neinhuis, 1997).
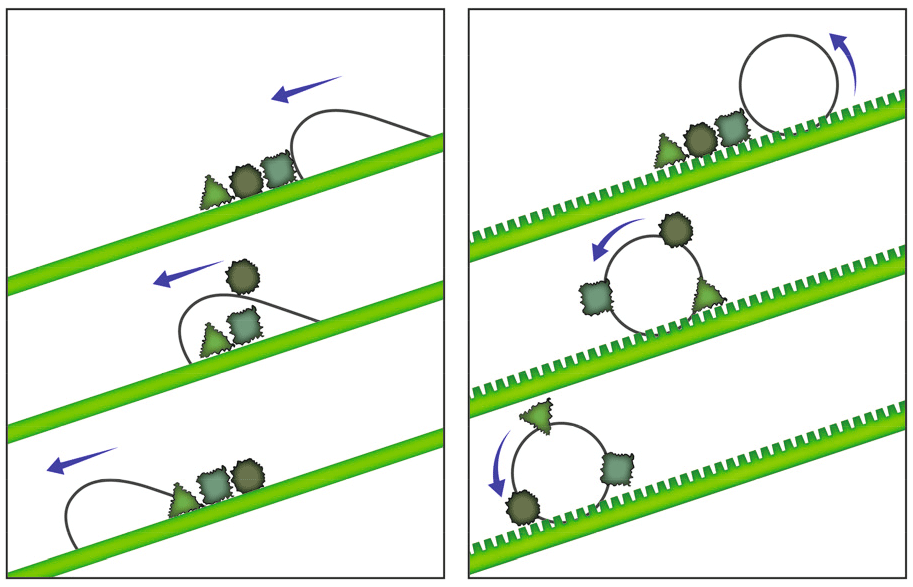
Wang et al. (2013) examined the seasonal changes in the amount of PM on leaf surfaces of three urban tree species during the growing seasons. They reported that PM accumulation increased from spring to autumn. This seasonal variation of PM loads is attributable to the increase in leaf surface wettability which the mechanical or chemical abrasion of leaf epicuticular wax layers might cause. Meanwhile, He et al. (2020) investigated the species-specific PM capturing capacity of evergreen species during the winter when the deciduous species lost their leaves and proposed the potential of evergreen species in winter.
In addition to leaves, different vegetative organs, such as branches and tree barks, can interact with PM and alleviate air pollution. Xu et al. (2019b) demonstrated that the PM mitigation capacity of leaf, branch, and bark surfaces tended to adsorb the highest proportion of large particles with diameters of 10-100 μm, and branch and epicuticular leaf wax had a greater tendency to encapsulate fine particles with diameters of 0.2-3.0 μm.
Relationship between Leaf Traits and PM Accumulation on Leaves
Interactive effects of different foliar traits could attribute to PM capture (Figure 4). The plant species with smaller leaves showed significantly higher mean PM densities on leaf surfaces (Weerakkody et al., 2017). Coniferous species with needle leaves have been reported as effective PM filters compared to broadleaved species (Song et al., 2015). A more turbulent flow could occur across conifer needles relative to broadleaves, resulting in a reduced thickness of the boundary layer in needle leaves. A boundary layer is relatively stationary and roles as a barrier between the surrounding air and the leaf surfaces (Chen et al., 2017).
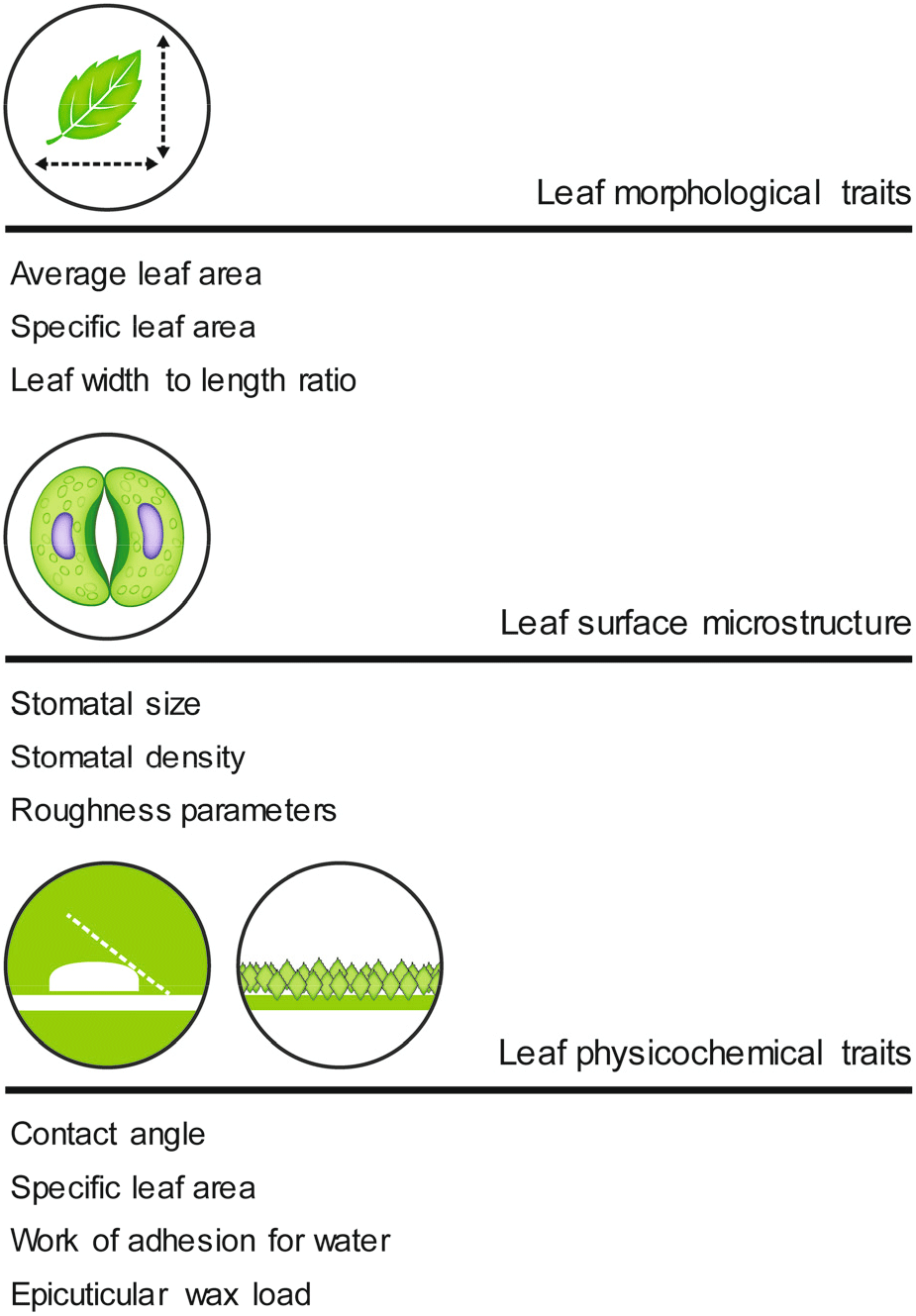
Moreover, the needle-leaved coniferous species are more efficient in removing PM2.5 than broadleaved species (Chen et al., 2017); however, within broadleaved species, leaf macro-morphology, such as leaf shape and venation pattern, don’t have a significant relationship with PM2.5 accumulations. Whereas Weerakkody et al. (2018) examined that the palmate-lobed leaves exhibited significantly higher PM densities in all particle size fractions (PM1, PM2.5, and PM10) than for both elliptical and linear-shaped leaves. Regarding leaf micromorphology, plant species with trichomes on leaf surfaces have a higher capacity for PM accumulation than those with smooth leaves (Sæbø et al., 2012; Chen et al., 2017; Chiam et al., 2019; Kwak et al., 2019). In addition, leaf surface roughness had a positive correlation with the PM capturing capacity of leaves (Chen et al., 2017; Zhang et al., 2020); however, some previous studies reported that there was no relationship between the two factors (Sæbø et al., 2012; Wang et al., 2015; Kwak et al., 2020).
The PM not only adsorbs on leaf surfaces but also accumulates within cuticular wax layers of leaves (Dzierżanowski et al., 2011; Popek et al., 2013). The leaf surface is normally covered with a hydrophobic cuticle, which is coated by an epicuticular wax with considerable structural and chemical variability (Barthlott et al., 1998). Lipids called waxes are integrated and superimposed with cutin forming cuticles which are composite materials. Epicuticular waxes strongly affect the wettability of the leaf surface while intracuticular waxes play a role as a transpiration barrier of water (Koch and Ensikat, 2008). An epicuticular wax layers cover leaf surfaces with a variety of morphology, such as thin film, platelets, and tubules. Waxes are made up of a mixture of compounds.
Depending on the plant species (Figure 5), organ, or developmental stage, the amount, form, chemical composition, and constituents of waxes are varied (Prüm et al., 2012; Wang et al., 2014). PM containing organic pollutants (polycyclic aromatic hydrocarbons (PAH), polychlorinated dibenzo-p-dioxins and dibenzofurans (PCDD/Fs), etc.) can have lipophilic properties and penetrate the wax layer of leaf surfaces (Kaupp et al., 2000; Jouraeva et al., 2002; Dzierżanowski et al., 2011). Thus, depending upon the thickness, chemical composition, and structure of the epicuticular wax layer, the foliar accumulation of PM can vary among species (Popek et al., 2013). Wang et al. (2015) found that the epicuticular wax ultrastructures affect the PM adsorption ability of plants; it decreases in the order of films, platelets, and tubules. In tested species, the epicuticular wax load showed no significant relationship with the amount of PM adsorbed on the leaf surfaces and exhibited a moderate correlation (r = 0.79) with the quantity of fine PM (0.2-2.5 μm) in waxes (Dzierżanowski et al., 2011).
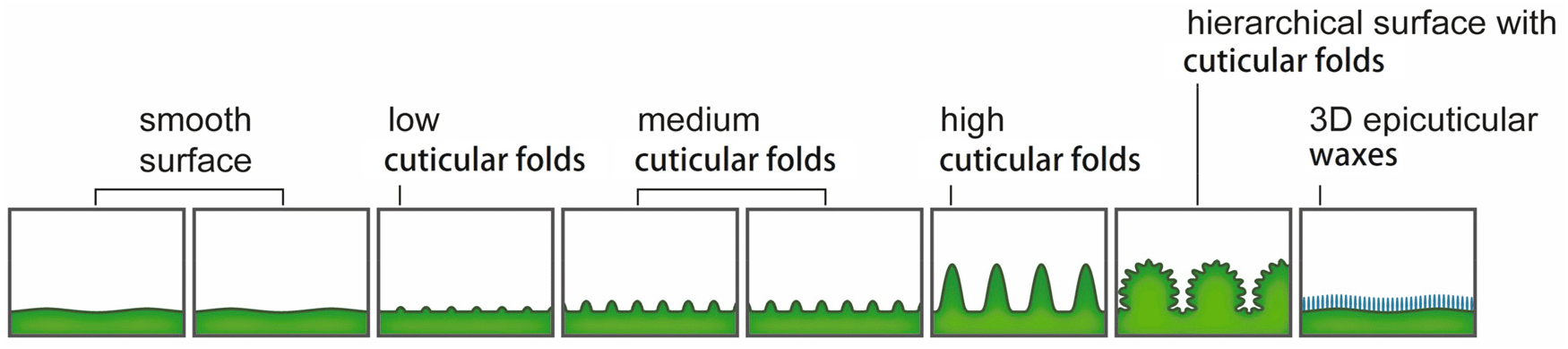
Conclusion
In this paper, we summarized the basic information of particle pollution, which is emerging as a problem with rapid industrialization and urbanization. Next, we reviewed various research that studied the potential of urban greening as a sustainable PM bio-filter. There are mainly studies on quantifying the amount of PM adsorbed to leaves, where PM is mainly collected in tree species used for urban greening, and further on the characteristics of various leaves affecting the PM capturing capacity. In addition to the quantitative analysis of PM adsorbed on leaves, some studies conducted the qualitative analysis of PM. Some studies examined the PM reduction effect on the forest scale rather than the individual level. More recent research examined the PM mobility from leaves after rainfall intensity and duration and seasonal changes of PM capturing capacity of tree species because the potential impacts of climate and season related to PM retention, PM resuspension, and PM wash-off cannot be neglected regarding urban greening. Through these evaluations, the interaction with the PM adsorption of leaves and the leaf traits was reviewed in detail by dividing the traits into morphological characteristics and physicochemical characteristics to provide the most basic data for planning urban greenery.