서 론
콩과(Fabaceae) 갯활량나물속(Thermopsis R. Br.)의 여러해살이풀인 갯활량나물[Thermopsislupinoides (L.) Link.]은 주로 바닷가 모래땅에 서식하며 5-8월에 노란색 나비모양 꽃이 총상꽃차례로 핀다. 초장은 40-80 cm 정도이며 3개의 소엽으로 이루어진 손바닥 모양 잎을 지닌다(Lee, 2014). 갯활량나물은 밥티시아(Baptisia australis (L.) R. Br.)와 형태가 유사하며 내건성이 강해 정원 소재로의 활용 가능성이 큰 식물이다. 또한 갯활량나물의 종자와 지상부는 루핀 알칼로이드 성분 때문에 전통적으로 소염제와 거담제, 구토제 등으로 사용되어 왔다(Saito et al., 1988; Yamazaki et al., 1994). 갯활량나물은 러시아와 일본, 북아메리카에 걸쳐 분포하며 한반도 내에는 강원도 이북에 자생한다(Lee, 2014). 남한에서는 강원도 양양군이 유일한 자생지로 현재 약 40개체만이 관측되고 있다.더욱이 최근 대규모 공사가 양양의 자생지 인근에서 진행되어 생육지 면적과 성숙개체 수의 급격한 감소를 보이고 있어 국가적색목록 위협(CR, Critically Endangered) 범주로 지정된 상태이다(Korea National Arboretum, 2021). 또한 강원도 양양군이 분포의 남방한계선인 갯활량나물은 지구온난화가 진행될수록 그 분포지가 점차 북쪽으로 이동하여 결국 한반도 내에 자생지가 사라질 것으로 예측되는 북방계식물이다(Gantsetseg et al., 2020). 이와 같이 분포의 한계선에 서식하는 식물은 기후변화와 같은 급격한 환경 변화에 특히 취약하다고 알려져 있음에도 현재 갯활량나물의 보전과 자생지 보호 대책이 미흡하여 보전 방안 마련이 시급한 상황이다(Kong, 1998).
한편, 식물원은 식물 다양성 보전의 중추적인 역할을 담당하며, 특히 식물원 내 전시원은 다양한 미기후의 서식 환경을 갖추고 있어 여러 식물종의 현지외 보전 장소로 활용될 수 있다(Price et al., 2004; Smith, 2019; Thomas et al., 2022). 전시원을 활용한 효과적인 현지외 보전을 위해서는 보전 대상 식물의 최적 서식 환경을 고려하여 전시원 내에 알맞은 미기후 조건을 지닌 장소에 식물을 식재하여야 한다(Yoon, 2005). 이를 위해서는 광, 온도, 수분 등 환경요인에 따른 식물의 생리・생태적 반응에 대한 연구를 통해 식물의 최적 생육 조건을 구명하는 것이 필요하다(Larcher, 2003). 그 중 광조건은 식물의 에너지 공급원이자 생장, 발달을 제어하는 주요 요인이며, 식물은 자신이 처한 광환경에 따라 광합성을 최적화하기 위한 방향으로 진화해왔다(Kim, 2013; Folta and Carvalho, 2015; Paradiso and Proietti, 2022). 식물은 광도 수준 차이에 따라 광형태형성학적 반응과 생리생태학적 반응을 통해 주어진 광환경에 적응하는데 광합성 기구가 지닌 가소성을 넘어서면 생장 및 생리 활동이 저해되어 도태된다(Park, 2001; Kim, 2008). 따라서 식물 생육을 위한 최적 광환경에 대한 조사는 효과적인 현지외 보전을 위해 필수적이다.
그러나 현재 갯활량나물의 현지외 보전에 관한 연구로는 종자 휴면 유형 및 타파 방법 구명(Kondo and Takahashi, 2004)과 조직배양(Yamazaki et al., 1994) 분야만이 수행된 상태로 아직까지 생체 보전을 위한 최적 생육 조건에 대한 연구는 수행된 적이 없다. 따라서 본 연구에서는 갯활량나물이 다양한 광도 조건에 따라 변화하는 생장과 생리적 반응을 조사, 분석하여 최적 생육을 위한 광조건을 구명하여 효과적인 현지외 보전에 기여하고자 한다.
재료 및 방법
실험에 사용된 식물체는 발아 후 2개월간 생장한 실생개체이다. 국립DMZ자생식물원에서 4°C에 냉장 보관한 종자를 2023년 5월 온실에서 발아시켜 사용하였다. 발아한 개체는 한 달간 생육 후 마사토와 원예상토(1:2)를 이용한 지름 15 cm 화분에 이식하였으며 7월 초까지 온실 내에서 생육시킨 후 시험 포지로 이동하였다. 시험 포지는 국립DMZ자생식물원 내 위치한 W2,000 × D14,000 × H2,000 규격의 plot 3 곳에 두었다. 저면관수를 통해 화분 내 토양이 마르지 않도록 관리하였다. 2023년 7월부터 9월까지 3개월간 서로 다른 광환경 수준에서 자라도록 하고, 성숙한 개체들의 생리적 차이를 조사하였다.
차광 처리 수준에 따른 생육환경 차이를 비교하기 위해 대조구 및 차광 처리구 내부에 온습도데이터로거(HOBO U23 Pro v2 U23-001; Onset; Boume, MA, USA)를 설치하여 대기 온습도를 조사하였다. 광량자센서(Quantum Sensor, Apogee, USA)를 데이터로거(MSTL Mini Logger, STA Corp., Korea)에 연결하여 광도를 조사하였으며, 휴대용 광파장 측정기(LI-180, Li-Cor Inc., USA)를 통해 광질을 측정하였다.
차광 처리를 위해 이중직 비닐 차광막을 이용하여 0%(이하 대조구), 50%, 75%, 95%의 차광처리구를 조성하였다. 실험 개체는 각 처리구당 6반복으로 배치하여, 차광 실험을 위해 총 24개체를 배치하였다. 처리구 내 환기를 위해 차광막 하부를 지면에서부터 20 cm 띄워 공간을 주었다.
차광 수준에 따른 갯활량나물의 엽생장특성을 알아보고자 처리구별 6장의 건실한 잎을 채취하여 엽면적당 건중량(Leaf mass per area; LMA)을 측정하였다. 엽면적은 엽면적 측정기(LI-3100C, Li-Cor Inc., USA)를 이용하여 측정하였고, 측정한 잎을 송풍식 건조기에 48시간 동안 80°C로 건조시킨 후 전자저울을 사용하여 건중량을 측정하였다. 엽면적당 건중량은 다음 식 1을 사용하여 산출하였다.
엽록소 함량을 조사하기 위하여 처리구별 3장의 잎을 채취한 후 잎 당 0.72 cm2의 엽편을 5 ml의 DMSO (Dimethyl sulfoxide) 용액이 들어있는 Vial에 넣어 6시간 동안 65°C의 항온기에서 색소를 추출하였다(Hiscox and Israelstam, 1979). 추출한 용액은 분광광도계(UV/VIS Spectro photometer-2550, Shimadzu Inc., Japan)를 통해 665.1 nm, 649.1 nm의 파장에서 흡광도를 측정하였다. 엽록소 a, b의 함량은 Wellburn(1994)의 식 2에 따라 산출하였다.
최대양자수율(Fv/Fm)과 비광화학적 형광소멸(Non-Photochemical Quenching; NPQ)의 측정을 위해 엽록소 형광반응 측정기(PAM-2100, Heinz Walz GmbH, Germany)를 이용하였으며, 처리구별 평균적인 생장을 보이는 5개체를 선정하여 진행하였다. 측정 전 sample clip으로 약 20분간 잎 측정 부위에 광을 차단하는 암적응을 진행하여 광합성계 열방출경로를 불활성시켰다. 형광 강도 측정은 200초 동안 측정광과 섬광을 순차적으로 비추는 Quenching analysis 방법을 이용하였다. 엽록소 형광 반응을 측정할 때 Fo는 0.1 μmol・m−2・s−1 이하의 광을, Fm은 0.8초 동안 8,000 μmol・m−2・s−1의 포화광을 조사하였다. 광화학 반응에 대한 값은 Lichtenthaler et al.(2005)의 공식을 사용하여 산출하였다.
광합성 특성을 조사하기 위하여 처리구별로 평균적인 생장 상태를 보이는 5개체를 선정하였다. 측정 하루 전에 수분 스트레스로 인해 생기는 광합성 편차를 방지하기 위해 관수를 진행하였으며, 검은 비닐을 씌워 암적응을 시켜 광에 의한 오차를 방지하였다. 휴대용 광합성 측정기(LI-6400XT, Li-Cor Inc., USA)를 사용하여 광-광합성곡선(Light Curve)과 CO2-광합성곡선(A-Ci Curve)을 작성하였으며 이에 대한 해석은 Kim and Lee(2001a)의 방법을 따랐다.
광-광합성곡선을 작성하기 위하여 PPFD (Photosynthetic Photon Flux Density)를 0, 25, 50, 75, 100, 150, 300, 500, 700, 1000, 1500 μmol·m−2·s−1로 조절하였으며, 유입 공기의 CO2 농도는 400 μmol·CO2·m−2·s−1 air로, 챔버 내 온도는 온도 20°C로 유지하여 광합성을 측정하였다. 광-광합선 곡선을 바탕으로 암호흡속도(Dark respiration; Rd)와 순양자수율(Apparent quantum yield; AQY), 광보상점(Light compensation point; LCP), 광포화점(Light saturation point; LSP), 최대광합성속도(Maximum photosynthetic rate; PNmax)를 산출하였다.
CO2-광합성곡선을 작성하기 위하여 대기 중 CO2 농도를 0, 50, 100, 200, 300, 400 μmol·CO2·m−2·s−1 air로 조절하였으며, PAR는 1,000 μmol·m−2·s−1로 유지하여 광합성과 엽육 내 CO2 농도(Ci)를 측정하였다. 이를 바탕으로 광호흡속도(Photo-respiration; Rp)와 CO2 보상점(CO2 Compensation point; CCP), 탄소고정효율(Carboxylation efficiency; CE)을 산출하였다.
기공전도도와 증산속도는 von Caemmerer and Farquhar (1981)의 식으로, 수분이용효율은 아래 식 3을 통해 산출하였다(Kim et al., 2002).
결 과
차광 처리에 따른 갯활량나물의 생장 및 생리적 특성을 관찰하기 위하여 전광 조건인 대조구와 50%, 75%, 95% 차광 처리구를 조성하였다.
온습도데이터로거(HOBO U23 Pro v2 U23-001; Onset; Boume, MA, USA)를 이용하여 처리구별 대기 중 온습도를 측정한 결과는 Table 1과 같았다. 실험 기간 동안 낮과 밤의 평균기온은 대조구에서 21.63/17.32°C로 가장 높았으며, 75% 차광 처리구에서 20.27/16.61°C로 가장 낮았다. 일 최고기온은 대조구에서 34.55°C로 가장 높았으며 최저기온은 55% 차광 처리구에서 2.61°C로 가장 낮았다. 반면 상대습도는 95% 차광 처리구에서 91.44%로 가장 높았으며, 대조구에서 86.51%로 가장 낮은 수치를 보였다(Table 1).
차광막 설치에 따른 실제 광도 차이를 비교하기 위해 광량자센서(Quantum Sensor, Apogee, USA)를 사용하여 광도를 조사하였다(Table 2). 50% 차광 처리구의 전광 조건에 대한 상대광도는 50.9%, 75% 차광 처리구는 26.2%, 95% 차광 처리구는 12.0%로 조사되어 95% 차광막의 경우 시판 기준에 비해 실제 광도 수준이 7% 높은 것으로 측정되었다.
차광막 내 R/FR 차이를 비교하기 위해 휴대용 광파장 측정기(LI-180, Li-Cor Inc., USA)를 이용하여 광질을 측정하였다(Table 2). 대조구와 50% 차광 처리구에서는 R/FR이 1.1이었으며, 75%와 95% 차광 처리구에서는 1.0으로 값의 유의한 차이를 보이지 않았다.
차광 수준에 따른 갯활량나물의 LMA를 측정하였다(Table 3). 갯활량나물은 차광 처리구에 비해 전광 조건인 대조구에서 LMA가 6.38±0.7 mg·cm−2로 유의하게 높은 값을 보였다. 갯활량나물은 차광 처리 수준이 높아질수록 LMA가 감소하는 경향을 보였지만 차광 처리간 유의성은 보이지 않았다.
Differences between groups were tested with Scheffe’s significant difference test or, when variances were unequal, with Dunnnett’s T3 test. Statistical significance was set at p < 0.05 and analyses were performed using SPSS (version 21). Values are means ± SD (n=6).
차광 수준이 갯활량나물의 엽록소 함량에 미치는 영향을 알아보기 위해 대조구와 처리구 별로 엽록소 함량을 조사하였다(Table 3). 차광 수준에 따른 갯활량나물의 엽록소 a, b 함량 및 엽록소 a+b 함량은 모두 50% 처리구에서 유의하게 높았으며, 전광 조건인 대조구에서 가장 낮은 값을 보였다. 차광 수준이 높아질수록 엽록소 a, b, a+b 함량이 감소하는 경향을 보였지만 차광 75%와 95% 처리구에서는 서로 유의미한 차이가 나타나지 않았다. 엽록소 a/b는 차광 수준에 따른 유의성을 보이지 않았다.
차광 수준에 따른 갯활량나물의 Fv/Fm(최대양자수율)과 NPQ(비광화학적 형광소멸)를 조사하였다(Figure 1 and Table 4). Fv/Fm은 75% 차광 수준에서 0.758±0.020으로 가장 낮았으며, 95% 차광 수준에서 0.813±0.010로 유의하게 높았다. NPQ는 75% 차광 수준에서 가장 높았지만 처리구별 유의성을 보이지는 않았다.
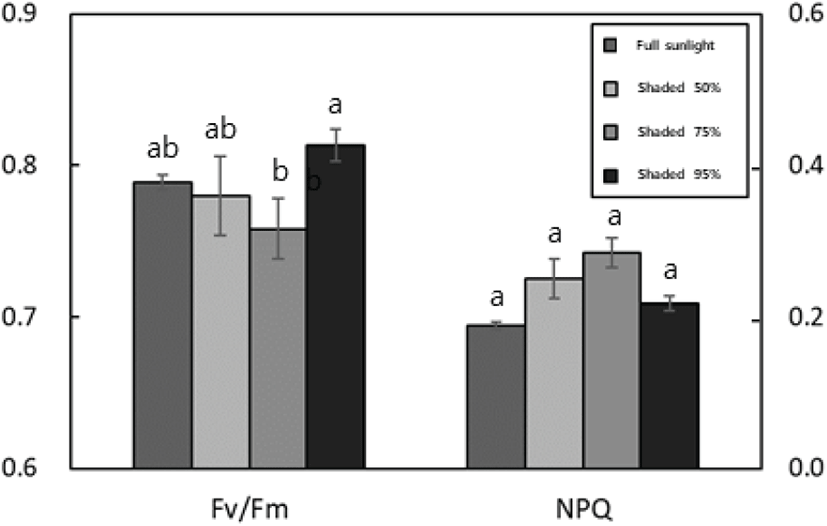
Differences between groups were tested with Scheffe’s significant difference test or, when variances were unequal, with Dunnnett’s T3 test. Statistical significance was set at p < 0.05 and analyses were performed using SPSS (version 21). Values are means ± SD (n=6).
차광 수준에 따른 갯활량나물의 광합성 반응을 비교하기 위해 광-광합선곡선을 작성하였으며 이를 바탕으로 암호흡속도(Rd)와 순양자수율(AQY), 광보상점(LCP), 광포화점(LSP), 최대광합성속도(PNmax)를 조사하였다[Figure 2(a) and Table 5]. 암호흡속도는 50% 차광 수준에서 0.74± 0.18 μmol CO2·m−2·s−1로 유의하게 낮았으며 차광 수준이 높아질수록 증가하는 경향을 보였다. 광보상점은 암호흡속도와 비슷한 경향을 보였는데 95% 수준에서 유의하게 높았으며 차광 수준이 50%까지 낮아짐에 따라 감소하는 경향을 보였다. 광포화점은 50% 차광수준에서 높고 전광 조건인 대조구에서 낮은 값을 보였지만 처리구 별 유의한 차이를 나타내지 않았다. 최대광합성속도는 50% > 75% > 00% > 95% 순으로 높았으며 차광 수준 별로 유의한 차이를 보였다. 순양자수율의 경우 50% 처리구에서 0.05 ±0.00 mmol CO2·mol−1로 가장 높았으며, 95% 처리구에서 0.025±0.00 mmol CO2·mol−1로 유의하게 낮았다.
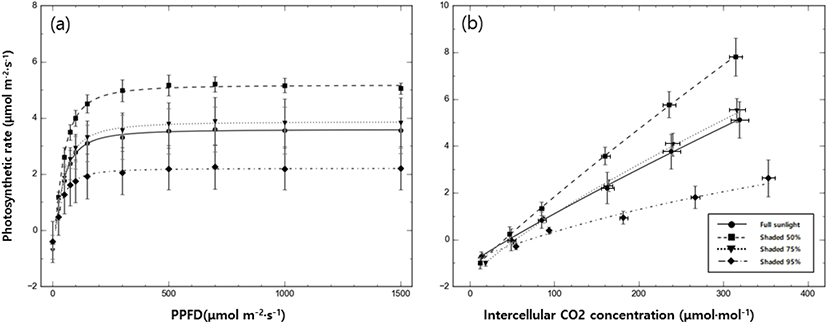
Dark respiration; Rd [μmol (CO2) m−2 s−1], Light compensation point; LCP [μmol m−2 s−1], Light saturation point; LSP [μmol m−2 s−1], Maximum photosynthesis rate; PNmax [μmol (CO2) m−2 s−1], net apparent quantum yield; AQY [mmol (CO2) mol−1], Photo-respiration rate; Rp [μmol (CO2) m−2 s−1], CO2 compensation point; CCP [μmol (CO2) m−2 s−1], Carboxylation efficiency; CE [μmol (CO2) mol−1]. Differences between groups were tested with Scheffe’s significant difference test or, when variances were unequal, with Dunnnett’s T3 test. Statistical significance was set at p < 0.05 and analyses were performed using SPSS (version 21). Values are means ± SD (n=5).
차광 수준의 차이가 엽육 내 CO2 농도(Ci)에 따른 갯활량나물의 광합성 반응에 미치는 영향을 조사하기 위해 CO2-광합선곡선(A-Ci Curve)을 작성하였으며 이를 바탕으로 광호흡속도(Rp)와 CO2 보상점(CCP), 탄소고정효율(CE)을 산출하였다[Figure 2(b) and Table 5]. 광호흡속도는 50% 차광처리에서 유의하게 높았으며 95% 차광처리에서 가장 낮았다. 탄소고정효율 또한 광호흡속도와 비슷하게 50% 차광처리에서 0.03±0.00 μmol CO2·mol−1으로 유의하게 높았으며 95% 차광처리에서 0.01±0.00 μmol CO2·mol−1로 가장 낮았다. CO2 보상점은 탄소고정효율과 반대 경향을 보였으며 50% 차광처리에서 가장 낮고 95% 차광처리에서 유의하게 높았다.
차광 수준에 따른 갯활량나물의 기공전도도, 증산속도, 수분이용효율을 조사하였다(Figure 3). 기공전도도와 증산속도의 경우 모두 대조구에서 가장 값이 낮았고 50% 차광 수준에서 가장 높았으며 75%와 95% 차광 수준은 비슷한 값을 보였다. 수분이용효율은 95% 차광 수준에서 현저히 낮은 값을 보였고 대조구에서 가장 높았으며 50%, 75% 차광 수준에서 비슷한 값을 보였다.
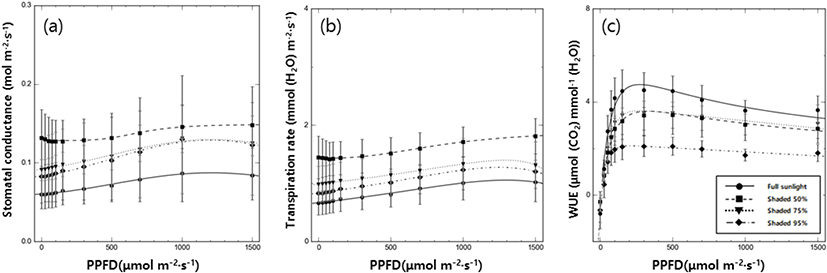
고 찰
대기 중 온도는 차광 수준이 높아질수록 감소하는 경향을 보인 반면 상대습도는 증가하였는데 이는 차광 수준이 높아질수록 차광막 내 대기가 직사광선의 영향을 적게 받아 기온 상승 폭이 적었고 증산 작용 또한 저하되어 상대습도가 높아진 영향으로 판단된다.
임분에서 임관을 거쳐 임상에 도달하는 빛은 전청광에 비해 광도가 저하되며 상층부에서 적색광 파장이 흡수되어 R/FR 비율 또한 달라져 식물 생장에 영향을 끼치는 것으로 알려져 있다(Skálová et al., 1999). 본 실험에서는 처리구간의 유의한 차이를 보이지 않아 광질이 식물에 미치는 영향이 없을 것으로 판단된다.
식물은 주어진 광조건에서 광합성을 최적화하기 위해 자신의 기관, 구조에 대한 물질 분배에 변화를 주는 광형태형성학적 적응 반응을 보인다(Kim et al., 2008; Folta and Carvalho, 2015; Paradiso and Proietti, 2022). 특히 동화기관인 잎의 형태적 특성은 광합성, 호흡 등과 큰 연관성을 보이는데(Chim and Han, 2003), 그 중 LMA는 엽의 단위 면적 당 무게로 식물의 수광도와 생장 및 탄소 획득의 관계를 설명해준다(Cheng et al., 2014). 광형태형성학적 측면에서 식물은 낮은 광도 조건이 주어졌을 때 수광량을 높이기 위해 잎의 두께는 줄어들고 엽면적은 넓어져 LMA가 감소하는 반응을 보인다(Salisbury and Ross, 1992). 본 실험에서 갯활량나물은 차광 수준이 높아짐에 따라 LMA가 감소하는 광형태형성학적 적응 반응을 보였으며, 이는 광수용능력의 극대화를 위해 엽면적이 증가하는 방향으로 탄소를 분배한 것으로 보인다(Song et al., 2016; Lee et al., 2012; Kim et al., 2020).
식물은 자신이 처한 광환경에 따라 엽록소 함량과 엽록소 형광 반응, 광합성 특성 등의 생리적 특성 또한 조절하여 광합성을 최적화는데 이를 생리생태학적 적응 반응이라고 한다(Kim et al., 2008; Paradiso and Proietti, 2022). 총엽록소 함량은 광합성 능력을 나타내는 생리적 지표 중 하나로 광조건에 따른 엽록소 함량은 식물의 내음성 수준에 영향을 받는다(Kim et al., 2001; Verhoeven et al., 2005; Mauro et al., 2011). 내음성이 높은 식물종은 일반적으로 내음성이 낮은 식물종에 비해 저광도 조건에서 광 사용 효율과 탄소 획득을 증가시키기 위해 높은 엽록소 함량을 나타낸다(Valladares and Niinemets, 2008). 하지만 내음성이 높은 식물종은 고광도 조건 하에서 광저해 현상을 방지하기 위해 광계II에 의한 빛 흡수를 감소시키는 광보호 작용으로 인해 총 엽록소 함량이 감소한다(Kyparissis et al., 2000; Je et al., 2006). 갯활량나물은 차광 50% 수준에서 가장 높은 총 엽록소 함량을 보였으며 차광 수준이 증가함에 따라 엽록소 함량이 감소하였다. 이는 갯활량나물의 낮은 내음성으로 인해 저광도 조건에서 생리생태학적 적응 반응이 원활하지 못했던 것으로 보인다. 하지만 전광 조건에서 갯활량나물이 총 엽록소 함량이 감소한 것으로 보아 과도한 광조건과 높은 평균 기온으로 인해 광저해가 일어남을 알 수 있다.
엽록소 a/b는 잎의 노화 및 음엽화의 지표로 활용되는 값으로, 내음성이 높은 식물의 경우 일반적으로 낮은 광도의 환경일수록 엽록소 a/b가 감소한다(Fails et al., 1982; Lee et al., 2019). 이는 음지식물이 저광도 조건에서 광수용능력을 극대화하는 방법의 일환으로 반응중심에 주로 위치한 엽록소 a가 광수확복합체 결합 단백질 안정화에 기여하는 엽록소 b로 전환되기 때문이다(Kim et al., 2003; Kim et al., 2020). 또한 이는 군락 상층부의 엽록소 a와 달리 단파장의 빛을 주로 흡수하는 엽록소 b의 양을 증가시켜 군락 하부에서의 빛 흡수 효율을 높이는 적응 반응이다(Fails et al., 1982; Kim and Lee, 2001b). 하지만 갯활량나물에서는 차광 수준별로 유의한 차이를 보이지 않았으며, 이는 차광 처리 수준에 따른 광질의 차이가 없었기 때문으로 판단된다.
광합성 과정은 식물이 처한 스트레스 수준에 민감하게 반응한다(Maxwell and Johnson, 2000). 특히 엽록소가 양자를 과도하게 흡수하여 여기상태(excited state)가 되었을 때 불안정한 상태의 해소를 위해 에너지 소멸 경로를 작동하는데, 이 때 발생하는 열과 형광 반응은 식물의 환경 스트레스를 나타내는 주요 지표로 사용된다(Maxwell and Johnson, 2000; Rascher et al., 2000). 엽록소 형광 반응 측정을 통해 얻게 되는 매개변수 중 최대양자수율(Fv/Fm)과 비광화학적 형광소멸(NPQ)을 스트레스 평가에 주로 사용한다(Strauss et al., 2006; Baker, 2008). Fv/Fm은 암적응 처리된 식물의 1차 광화학 최대 양자 수율을 나타내며 광계II 활성과 광합성 능력을 의미하고, 일반적으로 식물이 받는 스트레스 정도가 증가함에 따라 감소하는 경향을 보인다(Baker, 2008; Borkowska, 2002; Lawson et al., 2008). NPQ는 빛 에너지를 받아 들뜬 엽록소가 열을 통해 방출하는 에너지 정도를 의미하며 일반적으로 스트레스 조건에서 증가한다(Genty et al., 1990). 갯활량나물의 경우 차광 수준이 증가함에 따라 Fv/Fm가 감소하는 경향을 보이다가 95% 차광 수준에서 가장 높을 값을 보였으며 NPQ는 이와 반대 경향을 보였다. 95% 차광 수준 처리구의 개체는 엽록소 형광 반응 조사 시 상부 잎들이 모두 떨어진 상태로 하부에 올라온 신엽을 대상으로 측정하였다. 이를 고려하였을 때 대부분의 잎들은 차광 스트레스로 인해 정상적인 생육을 하지 못하고 낙엽하였으며 비교적 건강한 신엽을 대상으로 측정을 진행하여 Fv/Fm이 높게 나온 것으로 보인다. Strasser et al.(2000)에 따르면 건강한 식물의 잎은 Fv/Fm이 0.8 정도를 보인다고 하는데 이와 일치하는 결과이다. 따라서 갯활량나물은 차광 수준이 높아짐에 따라 낮은 광도로 인해 Fv/Fm은 감소하고 NPQ는 증가하여 생육의 저하를 나타내는 것으로 생각되지만 정확한 비교를 위해선 95% 차광 수준 처리구가 낙엽하기 전에 차광 스트레스를 받은 잎의 엽록소 형광 반응을 측정한 데이터가 필요할 것으로 보인다.
식물은 호흡을 통해 생존과 생장에 필요한 에너지를 획득하는데, 이 과정을 나타내주는 지표로 암호흡속도(Rd)가 있다(Noguchi et al., 1996). 일반적으로 내음성이 강한 식물은 낮은 광도 조건 하에서 효율적으로 빛을 이용하기 위해 암호흡속도가 저하되어 광보상점이 낮아지며 높은 광합성률을 보인다고 알려져있다(Walters et al., 1993; Lee et al., 2013). 하지만 갯활량나물은 50% 차광 수준에서는 낮은 암호흡속도와 광보상점 값을 보이며 광합성률이 높았던 반면, 차광 수준이 높아질수록 암호흡속도와 광보상점이 증가하였으며 95% 차광 처리구에서 가장 높은 광보상점과 가장 낮은 광합성률을 보였다. 이는 갯활량나물이 낮은 내음성으로 인해 낮은 광도 하에서 광합성 능력이 저하된 것으로 보인다. 내음성이 강한 음지식물의 경우 광보상점과 광포화점이 각각 5-10 μmol·m−2·s−1, 100-200 μmol·m−2·s−1에 분포한다는 연구 결과와 비교하였을 때, 갯활량나물은 50% 차광 처리구에서는 낮은 광도에 적응하여 음지식물 수준의 광보상점을 보이는 생리생태학적 적응 반응을 나타냈지만 나머지 수준에서는 광보상점과 광포화점 모두 기준 이상의 값을 보여 생리생태학적 적응 반응이 원활하지 못한 것으로 판단된다(Larcher, 2003).
순양자수율이란 광도 변화에 따른 광합성률의 변화가 직선에 가까운 약광에서 광 에너지를 광화학적 에너지로 전환하는 효율을 의미한다(Evans, 1987). 순양자수율은 광합성 능력을 나타내는 지표로(Kim and Lee, 2001a), 갯활량나물의 경우 50% > 75% > 00% > 95% 순으로 광합성 능력과 일치하는 경향을 나타내었다. 갯활량나물은 대조구에 비해 50% 차광 수준에서 광호흡과 탄소고정효율이 높아지고 CO2 보상점은 낮아져 탄소고정계의 활성이 증가하는 생리생태학적 적응 반응이 나타났다. 하지만 차광 수준이 더 높아짐에 따라 탄소고정계의 활성이 감소하는데 이는 생육을 위한 최소 요구 광도 수준에 미치지 못했기 때문으로 보인다.
식물은 자신이 처한 환경에 적응하기 위해 기공 개폐 수준을 조절하여 대기와 물질 교환을 통제한다. 대기로의 수분 확산 정도를 나타내는 기공전도도를 통해 기공 개폐 정도를 확인할 수 있으며 이는 증산속도와 광합성, 수분이용효율에 영향을 미친다고 알려져 있다(Hinckley and Braatne, 1994; Lim et al., 2006; Lee et al., 2012). 갯활량나물은 50% 차광할 때 기공전도도와 증산속도가 높아 대기와의 물질 교환이 활발히 일어남과 동시에 수분이용효율도 높게 나타난 반면, 75%와 95% 차광 수준에서는 최소한의 광요구도 보다 낮은 광도로 인해 기공전도도와 증산속도와 같은 광합성 기능이 저하되는 것으로 보이며, 특히 95% 차광 수준에서는 수분이용효율 또한 현저히 감소하였다. 전광 조건인 대조구에서는 기공전도도의 감소로 증산으로 인한 수분 손실이 감소하여 수분이용효율의 증가가 나타난 것으로 보인다(Farooq et al., 2008; Lee et al., 2013).
차광 수준별 상대광도와 생장특성, 생리적 특성 간의 Pearson 상관분석한 결과를 Figure 4에 나타내었다. 갯활량나물의 개체 속성간 상관관계 분석 결과 상대광도와 LMA는 양의 상관을 보였다(p<0.001). 이는 낮은 광도 조건에서 잎의 수광량을 최대로 하기 위한 광형태형성학적 적응반응으로 보인다(Salisbury and Ross, 1992). 또한 엽록소 a+b 함량은 순양자수율(p<0.05), 최대 광합성 속도, 탄소고정효율(p<0.05)과 양의 상관을 나타내었는데, 이를 통해 엽록소 함량이 높을수록 광합성 활성의 증가를 보인다는 것을 알 수 있다. 최대 광합성 속도는 암호흡속도(p<0.01), 광보상점(p<0.001)과 음의 상관을, 순양자수율(p<0.001), 광포화점(p<0.05)과는 양의 상관을 보였다. 이는 식물이 주어진 광환경에서 순광합성량을 최대화하기 위해 암호흡속도와 광보상점을 낮추고 광포화점과 최대 광합성 속도를 높이는 반응과 일치한다(Walters et al., 1993; Lee et al., 2013). 탄소고정계의 경우, 탄소고정효율은 CO2 보상점(p<0.001), 광보상점(p<0.05), 암호흡 속도(p<0.01)와 음의 상관을 보이고 광호흡 속도, 최대 광합성 속도(p<0.01)와 양의 상관을 나타내었다. 탄소고정효율은 CO2 고정효소인 rubisco의 활성 및 함량을 나타내는 지표로, 본 연구의 결과는 탄소고정효율이 높을수록 광합성 기능이 향상되었다는 연구(Reddy et al., 2004)와 일치하며 rubisco가 증가함에 따라 O2의 고정도 증가하여 광호흡이 낮아지는 적응 반응을 보인 것으로 생각된다(Kim et al., 2008).
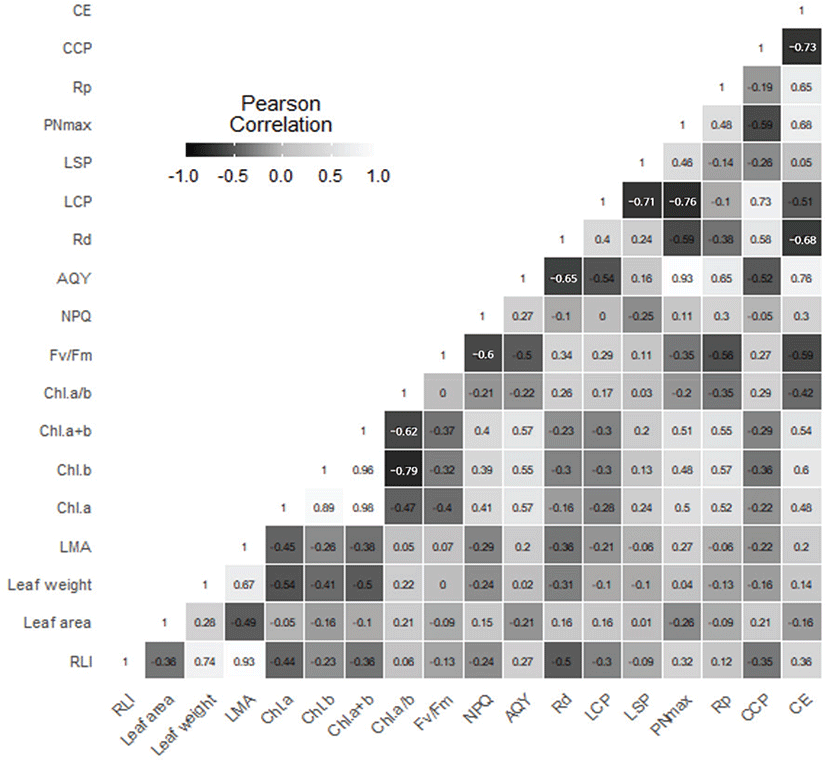
결 론
본 연구에서는 차광 수준에 따른 광환경의 차이가 갯활량나물의 생장 및 생리적 반응에 미치는 영향을 조사하기 위해 차광 처리에 따른 갯활량나물의 엽면적당 건중량, 엽록소 함량, 엽록소 형광 반응 및 광합성 특성을 측정하였다. 갯활량나물의 경우 차광 수준이 높아짐에 따라 엽면적이 증가하고 LMA가 감소하여 낮은 광도 조건에서 광형태형성학적인 적응을 보였다. 생리생태학적 적응 반응은 50% 차광 수준에서 엽록소 함량, 광포화점, 광합성률, 탄소고정효율, 기공전도도, 증산속도, 수분이용효율 등 전반적으로 광합성 기능이 양호했던 반면, 95% 차광 수준에서는 생육에 필요한 최소 광도 조건이 충족되지 못하여 광합성 기능이 크게 저하되는 것으로 나타났다. 이를 통해 갯활량나물의 생육에 있어서는 50% 차광 수준이 가장 적절한 것을 알 수 있었다. 본 연구 결과는 갯활량나물의 효과적인 현지외 보전을 위한 적지 선정 및 기반 조성을 위한 자료로 활용될 수 있을 것으로 기대된다.